Nitrous oxide’s powerful greenhouse effect stems from its unique molecular structure and extraordinary atmospheric persistence. You’ll find it has 265-310 times the warming potential of CO₂ over 100 years, with a remarkable 121-year average residence time. Its linear N≡N-O arrangement and permanent dipole moment enable significant infrared absorption, while its stability at lower altitudes prevents rapid breakdown. Primary removal occurs through O₃ reactions at 32 km altitude understanding these mechanisms reveals why N₂O remains a critical climate concern.
Chemical Structure and Properties
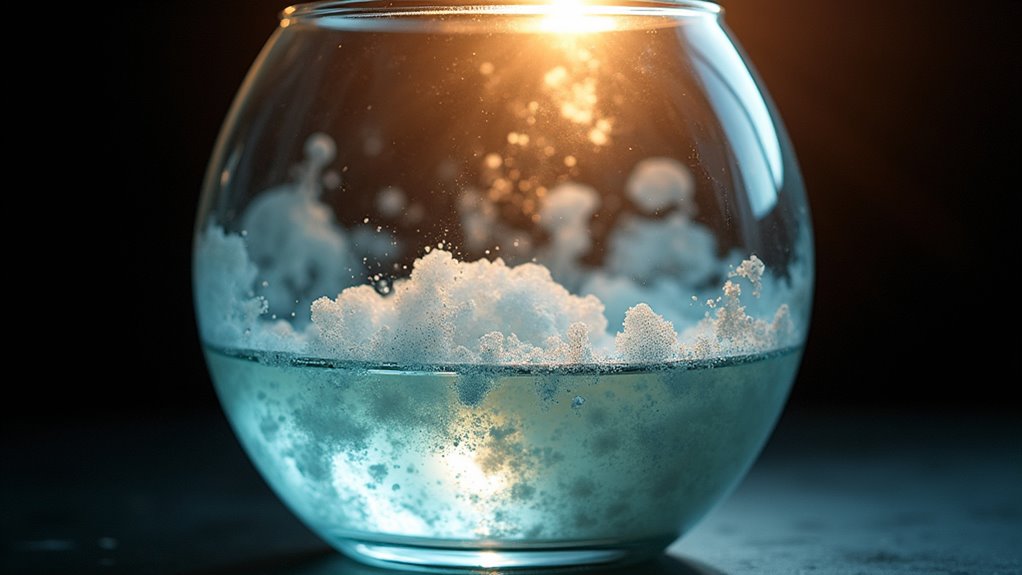
Nitrous oxide’s chemical structure features a linear molecular arrangement with two nitrogen atoms triple-bonded to each other and a single bond to oxygen (N≡N-O). The molecular bond strength is characterized by specific bond lengths: 119 pm for N-O and 113 pm for N-N. You’ll find this neutral molecule has a permanent dipole moment due to electronegativity differences between atoms. The molecule exhibits resonance bonding characteristics that contribute to its overall stability.
The chemical bonding stability of N₂O is maintained at room temperature, where it exists as a colorless, odorless gas with a molecular weight of 44.0128 g/mol. While it’s non-flammable, its chemical properties allow it to support combustion like oxygen. You’ll notice it has high water solubility compared to other gases and maintains a vapor density greater than air, contributing to its unique atmospheric behavior.
Global Warming Potential Analysis
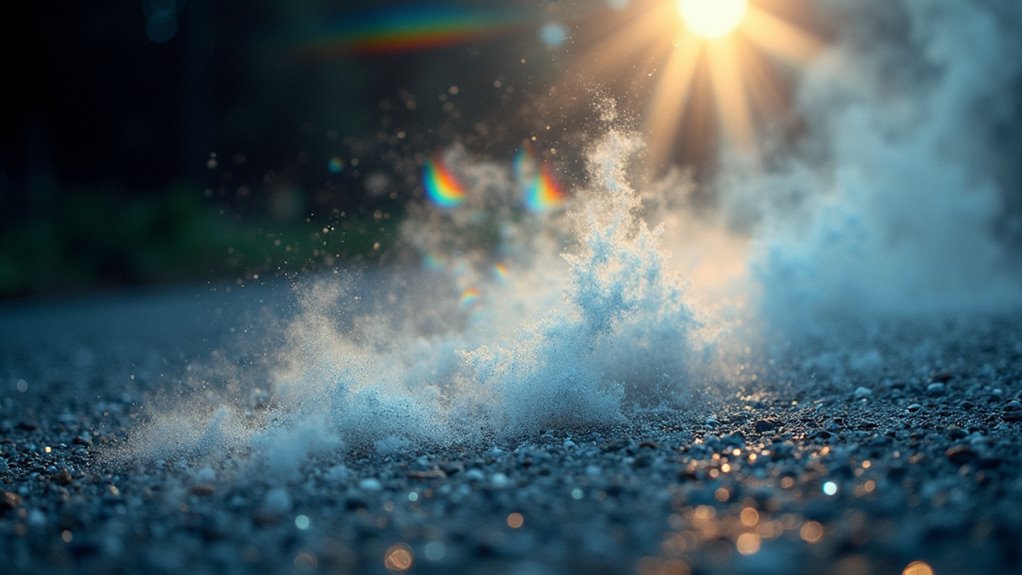
When analyzing greenhouse gas impacts, N₂O’s extraordinary global warming potential stands out at 265-310 times that of CO₂ over a 100-year period. You’ll find its potency stems from strong infrared absorption and persistent atmospheric lifespan, yielding significant emission distribution across sectors. With an atmospheric lifetime of 120 years, nitrous oxide’s effects on climate change are particularly long-lasting and concerning. Soil microorganisms are responsible for the majority of nitrous oxide production during nitrogen cycling in agricultural lands.
Time Horizon | GWP Value | Key Impact | Regional Mitigation Strategies |
---|---|---|---|
20-year | 280-310 | Near-term climate risks | Precision farming |
100-year | 265-310 | Policy standard | Nitrogen-use efficiency |
500-year | 170 | Long-term effects | Biochar application |
Annual Growth | 1.4 ppb | Temperature rise | Industrial abatement |
Current atmospheric N₂O levels increase by 1.4 ppb annually, potentially adding 0.35°C warming by 2100. Agriculture dominates emissions at 74%, while industrial processes and fossil fuel combustion contribute 6% and 10%, respectively. Meeting 2°C pathway goals requires a 21% reduction by 2030.
Atmospheric Residence Time
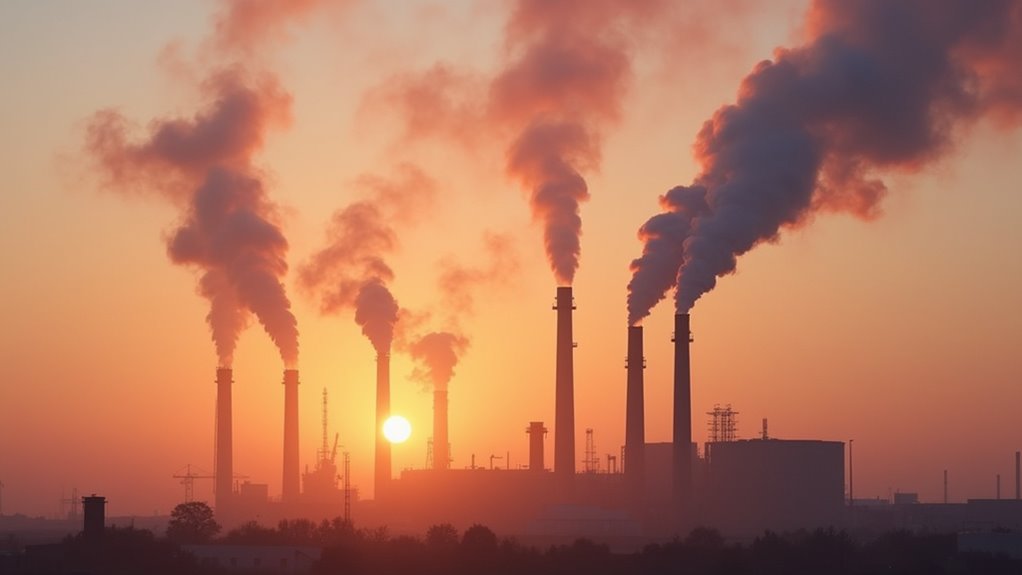
Nitrous oxide’s extended residence time of 121 years enables it to accumulate and trap heat in Earth’s atmosphere for over a century before decomposition through stratospheric processes. You’ll find N₂O’s persistence is influenced by feedback mechanisms that reduce its effective lifetime to 109 years, while its primary removal occurs through reactions with O₃ and photolysis in the stratosphere at altitudes near 32 km.
N₂O’s long atmospheric lifespan of 116 ± 9 years contributes to both its potent greenhouse effect and its role in stratospheric ozone depletion, making it particularly concerning for climate scientists studying long-term atmospheric changes.
Prolonged Heat-Trapping Effects
Consistently, atmospheric measurements reveal that N₂O maintains a remarkably long residence time of 121 years on average, with semi-empirical methods suggesting 116 ± 9 years based on satellite and laboratory data. You’ll find that stratospheric removal efficiency peaks at 32 km altitude, where O(1D) photolytic reactions serve as the primary sink mechanism.
The gas’s heat-trapping persistence is influenced by feedback effects on lifetime, with an autocatalytic loop that’s moderated by a feedback factor of 0.94 ± 0.01. This self-regulation has reduced the effective residence time from a preindustrial 123 years to approximately 109 years. Temperature-sensitive kinetics and solar irradiance variations in the tropical stratosphere continue to affect N₂O’s breakdown patterns, as confirmed by Aura MLS satellite measurements from 2005-2021.
Century-Long Decomposition Process
Through extensive atmospheric measurements and modeling, scientists have determined that N₂O maintains an average residence time of 116 ± 9 years, making it one of the longest-lasting greenhouse gases. Regional atmospheric modeling confirms that stratospheric transport pathways substantially influence N₂O’s decomposition process, with primary breakdown occurring at approximately 32 km altitude.
Effective residence time of 109 years accounts for N₂O’s self-regulating feedback mechanisms. Stratospheric loss rates vary with altitude and primarily occur through O(¹D) reactions. Temperature-dependent breakdown intensifies in upper atmospheric layers. Tropical regions show distinct variations in decomposition patterns. Current measurements indicate a 3% lifetime decrease between 2005-2021.
You’ll find this extended atmospheric persistence particularly consequential, as it enables N₂O to exert multigenerational climate impacts through sustained radiative forcing and gradual degradation processes.
Ozone Depletion Duration
Multiple atmospheric measurements reveal N₂O’s extraordinary persistence, with a baseline residence time of 116 ±9 years that’s validated through satellite and stratospheric data. EPA findings indicate a 121-year average, while preindustrial estimates suggest 123 years.
Recent stratospheric trends show a -2.1 ±1.2% lifetime decrease per decade (2005-2021). You’ll find that N₂O’s primary loss occurs at 32 km altitude through reactions with O(¹D) atoms, requiring molecular oxygen and ozone photolysis. Chemistry-transport models have confirmed these lifetime adjustments, though they’ve historically overestimated durations due to undervalued N₂O concentrations in the tropical upper stratosphere.
When accounting for feedback dynamics, the effective residence time reduces to 109 years, considerably impacting projections of future N₂O levels and their ozone-depleting potential.
Major Emission Sources Worldwide
N₂O emissions originate chiefly from agricultural activities, which account for 74% of anthropogenic sources in the 2010s. Decadal emission trends show significant increases in developing nations, while policy implementation challenges persist in balancing food production needs with emission controls. Recent analysis utilizes CO2-equivalent emissions to measure and compare the climate impact across different greenhouse gases. These emissions have experienced a 40% growth rate since 1980, highlighting the urgent need for mitigation strategies.
Agricultural activities dominate N₂O emissions, with developing nations showing rising trends amid challenges between food security and environmental protection.
Key emission sources include:
- Synthetic fertilizer application, triggering soil microbial processes
- Livestock waste decomposition and management systems
- Industrial processes, particularly nitric acid production
- Fossil fuel combustion in vehicles and power plants
- Natural sources, contributing 60% of global baseline emissions
You’ll find the highest emissions concentrated in ten nations, with China, India, and the U.S. leading global outputs. While European countries have successfully reduced emissions through regulations, developing nations continue struggling with agricultural expansion demands and industrial growth.
Environmental Impact Assessment
N₂O’s atmospheric degradation occurs primarily in the stratosphere through photochemical reactions with ozone (O₃), converting it into nitrogen oxides (NOₓ) that further catalyze ozone depletion.
Without immediate intervention to reduce emissions, scientists warn that N₂O is now the leading ozone-depleter being released into the atmosphere.
You’ll find that N₂O’s 114-year atmospheric lifetime means its cumulative emissions persist and contribute to a warming potential 270 times greater than CO₂ over a 100-year period.
The compound’s long-term ecosystem effects manifest through increased UV radiation exposure due to ozone depletion, while simultaneously intensifying the greenhouse effect by absorbing infrared radiation in specific atmospheric windows between 7-8 μm wavelengths.
Agriculture is a significant contributor to this problem, with soil management practices generating nearly three-quarters of U.S. nitrous oxide emissions.
Since 1980, human-made emissions have shown alarming increases primarily due to agricultural activities and fertilizer use.
Atmospheric Degradation Mechanisms
Understanding atmospheric degradation mechanisms requires examining the complex photochemical and chemical processes that break down nitrous oxide in the stratosphere. The degradation occurs through multiple catalytic decomposition pathways and adsorption desorption dynamics that affect its persistence as a greenhouse gas.
N2O undergoes photolysis when UV radiation breaks molecular bonds, converting it into N2 and reactive oxygen species. Surface-mediated reactions accelerate decomposition through heterogeneous catalysis on aerosol particles. Chemical reactions with excited oxygen atoms (O(1D)) serve as the primary removal mechanism in the stratosphere. With atmospheric concentrations increasing by one part per billion annually, the degradation processes struggle to keep pace with emissions. Selective catalytic reduction using noble metal catalysts provides an efficient artificial decomposition route. Thermal decomposition occurs at temperatures above 600°C, though this process is less significant in atmospheric conditions. Metal oxide catalysts can amplify N2O breakdown through electron transfer mechanisms, particularly in the presence of sunlight. The process of decomposition is often strongly climate-dependent, making its breakdown efficiency vary considerably under different environmental conditions.
Long-Term Ecosystem Effects
While nitrogen cycle disruptions manifest across diverse ecosystems, the long-term environmental impacts of N₂O emissions present complex challenges for biodiversity and ecological stability. Warmer temperatures accelerate the release of nitrous oxide from soils, creating a dangerous climate feedback loop. Agricultural activities drive approximately 60% of human-caused N₂O emissions. This gas is colorless and tasteless, making it difficult to detect during atmospheric monitoring.
You’ll observe nutrient cycling disruption most prominently in temperate zones, where studies show a 55% decline in N₂O response over 23 years of persistent nitrogen amendments. This altered biogeochemistry triggers vegetation community shifts, particularly affecting plant-fungal relationships through eutrophication and acidification.
Higher latitudes experience intensified N₂O releases, correlating with increased precipitation levels. In shrubland ecosystems, you’ll find the most dramatic emission responses to nitrogen inputs. The cascading effects extend beyond terrestrial systems excess nitrogen compounds deteriorate water quality, creating oxygen-depleted zones that compromise aquatic biodiversity and ecosystem functionality. These impacts persist due to N₂O’s 100+ year atmospheric lifetime.
Ozone Layer Interactions
Deep within the stratosphere, nitrous oxide initiates complex chemical destruction pathways that systematically break down Earth’s protective ozone layer. Through photolysis and reactions with excited atomic oxygen (O(¹D)), N₂O converts to NO, triggering catalytic cycles that accelerate ozone depletion.
Stratospheric nitrous oxide launches destructive chemical cascades, converting to NO and systematically destroying Earth’s vital ozone shield.
While self-healing mechanisms partially offset these effects, increasing oceanic emission contributions through newly unearthed inorganic pathways continue to challenge recovery efforts. The impact of N₂O on ozone depletion is projected to decrease by 10-20% by 2100 compared to levels observed in the year 2000.
- N₂O accounts for approximately 30% of current stratospheric ozone loss
- Photolysis breaks N₂O into NO + O(³P), initiating ozone destruction
- ClOₓ/BrOₓ cycles interact with N₂O, affecting ozone hole dynamics
- Marine environments, especially upwelling zones, serve as N₂O hotspots
- N₂O’s 150-year atmospheric lifetime enables sustained stratospheric accumulation
These chemical processes, combined with N₂O’s persistence, make it a dominant force in ongoing ozone depletion.
Climate Change Acceleration Mechanisms
Through its exceptional molecular properties, nitrous oxide accelerates climate change at a rate 300 times more potent than CO₂ over a century-long timeframe. You’ll find N₂O’s radiative forcing impact of 0.17 W/m² intensifies climate system feedbacks, creating disproportionate warming impacts across the atmosphere.
The gas’s molecular structure efficiently absorbs infrared radiation at 7.8 μm wavelengths, while its 114-year atmospheric lifespan guarantees prolonged heating effects. You’re witnessing a 40% surge in global emissions since 1980, with current levels exceeding pre-industrial concentrations by 30%. Agricultural activities drive 74% of anthropogenic emissions, while fossil fuels contribute 12%. This combination of high GWP, extended atmospheric residence, and increasing emission rates amplifies N₂O’s role in accelerating global temperature rise.
Mitigation and Control Measures
Effective N₂O mitigation strategies encompass multiple integrated approaches targeting agricultural emissions, with fertilizer management at the forefront. Through careful cost benefit analysis and stakeholder engagement, you’ll find significant emission reductions across these interventions:
- Nitrification inhibitors slash N₂O emissions up to 80% by suppressing microbial ammonium conversion
- High-temperature biochar applications reduce emissions by up to 80% while improving soil quality
- Arbuscular mycorrhizal fungi advance nutrient uptake, cutting emissions by 75%
- Precision irrigation and fertilizer timing minimize excess nitrogen by 30-40%
- Integrated nutrient management combines multiple techniques for ideal results
You’ll achieve maximum impact by implementing thorough monitoring systems and pairing carbon sequestration with N₂O reduction strategies. These data-driven approaches enable real-time adjustments while delivering measurable climate benefits through strategic intervention combinations.
Frequently Asked Questions
How Does Nitrous Oxide Affect Human Health When Released Into the Atmosphere?
You’ll face serious health risks from heightened nitrous oxide levels through multiple pathways. As N₂O depletes stratospheric ozone, you’re exposed to 3-5% more UV radiation for every 1% ozone loss, leading to increased skin cancer and 200,000+ annual cataract cases.
You’ll also experience respiratory complications from N₂O-driven climate effects, which intensify wildfires and increase emergency room visits for breathing problems by 50%.
What Role Does Nitrous Oxide Play in Natural Ecosystems and Plant Growth?
In natural ecosystems, you’ll find nitrous oxide plays a pivotal role in nitrogen cycling, where it acts as an intermediate compound during soil bacterial processes. It’s produced through plant respiration and microbial activity, particularly during nitrification and denitrification.
The gas helps regulate soil nitrogen availability, with microorganisms converting NH4+ to NO3- at rates of 2-500 kg N ha−1 year−1, directly impacting plant nutrient uptake and growth patterns.
Can Nitrous Oxide Emissions From Soil Be Measured Accurately at Local Levels?
You can measure soil N2O emissions at local levels through several precise methods, though challenges exist. Static chamber systems capture microbial nitrous oxide production by monitoring concentration changes over time.
Modern analyzers detect soil nitrogen dynamics at parts-per-billion sensitivity. You’ll achieve most accurate results by combining automated chambers with laser spectroscopy, which provides real-time measurements of N2O flux rates at sub-hourly intervals.
How Do Different Weather Conditions Influence Nitrous Oxide Production and Release?
You’ll observe that weather conditions greatly control nitrous oxide emissions through two key pathways. When soil moisture levels reach 60-70% water-filled pore space, you’ll find ideal conditions for microbial activity.
Temperature directly affects emission rates – for every 10°C increase, you’ll typically see a 2-3x acceleration in microbial processes. During rainfall events, you’ll notice emissions can spike 3-5x higher in waterlogged soils compared to well-drained conditions.
What Alternatives Exist for Industries That Currently Depend on Nitrous Oxide?
You’ll find numerous alternative industrial processes replacing N2O across sectors. In automotive, you can implement electric vehicles and hybrid turbocharging systems, reducing emissions by up to 100%.
For medical applications, you’re able to utilize TIVA or regional anesthesia, cutting N2O usage by 80%.
Sustainable production methods include nitrogen-based dispensing systems for beverages and argon gas for electronics manufacturing, offering 95% lower global warming potential compared to N2O-based processes.